Caloric Restriction for anti-aging and longevity - does it work in non-obese humans?
Background
How does it work?
Calorie restriction – just another fancy name for dieting?
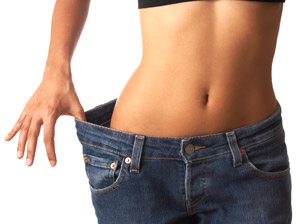
Drum roll… does chronic calorie restriction work in non-obese humans?
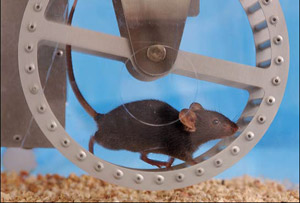
Adverse health effects of chronic calorie restriction in humans

What about the Okinawans?
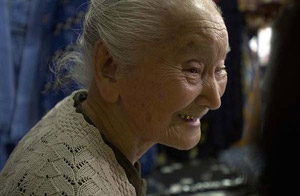
Living Fast, Dying When?

Does calorie restriction work by reducing intra-abdominal “belly” fat?
What about calorie restriction mimetics?
Exercise as a “human calorie restriction strategy”

Figure modified from Handschin, C., Caloric restriction and exercise "mimetics'': Ready for prime time? Pharmacol Res, 2016. 103: p. 158-66
Restricting calories vs. carbs
Conclusion
References:
1. McCay, C.M. and M.F. Crowell, Prolonging the life span. . Sci. Mon., 1934. 39: p. 405–414.
2. McCay, C.M., M.F. Crowell, and L.A. Maynard, The effect of retarded growth upon the length of life span and upon the ultimate body size. J Nutr Biochem, 1935. 10: p. 63-79.
3. Masoro, E.J., Caloric restriction and aging: an update. Exp Gerontol, 2000. 35(3): p. 299-305.
4. Weindruch, R. and R.S. Sohal, Seminars in medicine of the Beth Israel Deaconess Medical Center. Caloric intake and aging. N Engl J Med, 1997. 337(14): p. 986-94.
5. Smith, J.V., L.K. Heilbronn, and E. Ravussin, Energy restriction and aging. Curr Opin Clin Nutr Metab Care, 2004. 7(6): p. 615-22.
6. Sohal, R.S. and R. Weindruch, Oxidative stress, caloric restriction, and aging. Science, 1996. 273(5271): p. 59-63.
7. Sell, D.R., et al., Longevity and the genetic determination of collagen glycoxidation kinetics in mammalian senescence. Proc Natl Acad Sci U S A, 1996. 93(1): p. 485-90.
8. Masoro, E.J., M.S. Katz, and C.A. McMahan, Evidence for the glycation hypothesis of aging from the food-restricted rodent model. J Gerontol, 1989. 44(1): p. B20-2.
9. Cefalu, W.T., et al., Caloric restriction decreases age-dependent accumulation of the glycoxidation products, N epsilon-(carboxymethyl)lysine and pentosidine, in rat skin collagen. J Gerontol A Biol Sci Med Sci, 1995. 50(6): p. B337-41.
10. Sell, D.R., et al., The effect of caloric restriction on glycation and glycoxidation in skin collagen of nonhuman primates. J Gerontol A Biol Sci Med Sci, 2003. 58(6): p. 508-16.
11. Teillet, L., et al., Food restriction prevents advanced glycation end product accumulation and retards kidney aging in lean rats. J Am Soc Nephrol, 2000. 11(8): p. 1488-97.
12. Raffoul, J.J., et al., Caloric restriction and genomic stability. J Nutr Health Aging, 1999. 3(2): p. 102-10.
13. Chung, H.Y., et al., The inflammation hypothesis of aging: molecular modulation by calorie restriction. Ann N Y Acad Sci, 2001. 928: p. 327-35.
14. de Cabo, R., et al., An in vitro model of caloric restriction. Exp Gerontol, 2003. 38(6): p. 631-9.
15. Bergamini, E., et al., The role of macroautophagy in the ageing process, anti-ageing intervention and age-associated diseases. Int J Biochem Cell Biol, 2004. 36(12): p. 2392-404.
16. Weindruch, R., et al., Microarray profiling of gene expression in aging and its alteration by caloric restriction in mice. J Nutr, 2001. 131(3): p. 918S-923S.
17. Masoro, E.J., Hormesis and the antiaging action of dietary restriction. Exp Gerontol, 1998. 33(1-2): p. 61-6.
18. Rattan, S.I., Applying hormesis in aging research and therapy. Hum Exp Toxicol, 2001. 20(6): p. 281-5; discussion 293-4.
19. Fontana, L. and S. Klein, Aging, adiposity, and calorie restriction. JAMA, 2007. 297(9): p. 986-94.
20. Goldstein, D.J., Beneficial health effects of modest weight loss. Int J Obes Relat Metab Disord, 1992. 16(6): p. 397-415.
21. Pasanisi, F., et al., Benefits of sustained moderate weight loss in obesity. Nutr Metab Cardiovasc Dis, 2001. 11(6): p. 401-6.
22. Vidal, J., Updated review on the benefits of weight loss. Int J Obes Relat Metab Disord, 2002. 26 Suppl 4: p. S25-8.
23. Le Bourg, E. and S.I. Rattan, Can dietary restriction increase longevity in all species, particularly in human beings? Introduction to a debate among experts. Biogerontology, 2006. 7(3): p. 123-5.
24. Martin, B., et al., "Control" laboratory rodents are metabolically morbid: why it matters. Proc Natl Acad Sci U S A, 2010. 107(14): p. 6127-33.
25. Skalicky, M., E. Narath, and A. Viidik, Housing conditions influence the survival and body composition of ageing rats. Exp Gerontol, 2001. 36(1): p. 159-70.
26. Teillet, L., S. Gouraud, and B. Corman, Does food restriction increase life span in lean rats? J Nutr Health Aging, 2004. 8(4): p. 213-8.
27. de Grey, A.D., The unfortunate influence of the weather on the rate of ageing: why human caloric restriction or its emulation may only extend life expectancy by 2-3 years. Gerontology, 2005. 51(2): p. 73-82.
28. Harrison, D.E. and J.R. Archer, Natural selection for extended longevity from food restriction. Growth Dev Aging, 1989. 53(1-2): p. 3.
29. Phelan, J.P. and M.R. Rose, Why dietary restriction substantially increases longevity in animal models but won't in humans. Ageing Res Rev, 2005. 4(3): p. 339-50.
30. Weindruch, R. and E.J. Masoro, Concerns about rodent models for aging research. J Gerontol, 1991. 46(3): p. B87-8.
31. Partridge, L. and D. Gems, Mechanisms of ageing: public or private? Nat Rev Genet, 2002. 3(3): p. 165-75.
32. Hayflick, L., Biological aging is no longer an unsolved problem. Ann N Y Acad Sci, 2007. 1100: p. 1-13.
33. Holliday, R., Aging is no longer an unsolved problem in biology. Ann N Y Acad Sci, 2006. 1067: p. 1-9.
34. Kirkwood, T.B., Understanding ageing from an evolutionary perspective. J Intern Med, 2008. 263(2): p. 117-27.
35. Rattan, S.I., Theories of biological aging: genes, proteins, and free radicals. Free Radic Res, 2006. 40(12): p. 1230-8.
36. Holloszy, J.O., Mortality rate and longevity of food-restricted exercising male rats: a reevaluation. J Appl Physiol, 1997. 82(2): p. 399-403.
37. McCarter, R.J., et al., Physical activity as a factor in the action of dietary restriction on aging: effects in Fischer 344 rats. Aging (Milano), 1997. 9(1-2): p. 73-9.
38. Speakman, J.R. and C. Hambly, Starving for life: what animal studies can and cannot tell us about the use of caloric restriction to prolong human lifespan. J Nutr, 2007. 137(4): p. 1078-86.
39. Holloszy, J.O., The biology of aging. Mayo Clin Proc, 2000. 75 Suppl: p. S3-8; discussion S8-9.
40. Holloszy, J.O., Exercise increases average longevity of female rats despite increased food intake and no growth retardation. J Gerontol, 1993. 48(3): p. B97-100.
41. Martin, C.K., et al., Effect of calorie restriction on the free-living physical activity levels of nonobese humans: results of three randomized trials. J Appl Physiol, 2011. 110(4): p. 956-63.
42. Martin, C.K., et al., Effect of calorie restriction on resting metabolic rate and spontaneous physical activity. Obesity (Silver Spring), 2007. 15(12): p. 2964-73.
43. Redman, L.M., et al., Metabolic and behavioral compensations in response to caloric restriction: implications for the maintenance of weight loss. PLoS One, 2009. 4(2): p. e4377.
44. Weyer, C., et al., Energy metabolism after 2 y of energy restriction: the biosphere 2 experiment. Am J Clin Nutr, 2000. 72(4): p. 946-53.
45. Larsen, T.M., S. Toubro, and A. Astrup, Efficacy and safety of dietary supplements containing CLA for the treatment of obesity: evidence from animal and human studies. J Lipid Res, 2003. 44(12): p. 2234-41.
46. Li, M.D., Leptin and beyond: an odyssey to the central control of body weight. Yale J Biol Med, 2011. 84(1): p. 1-7.
47. Gautron, L. and J.K. Elmquist, Sixteen years and counting: an update on leptin in energy balance. J Clin Invest, 2011. 121(6): p. 2087-93.
48. Barter, P.J., et al., Effects of torcetrapib in patients at high risk for coronary events. N Engl J Med, 2007. 357(21): p. 2109-22.
49. Chapman, M.J., et al., Cholesteryl ester transfer protein: at the heart of the action of lipid-modulating therapy with statins, fibrates, niacin, and cholesteryl ester transfer protein inhibitors. Eur Heart J, 2010. 31(2): p. 149-64.
50. Dozmorov, I., et al., Gene expression profile of long-lived snell dwarf mice. J Gerontol A Biol Sci Med Sci, 2002. 57(3): p. B99-108.
51. Ikeno, Y., et al., Delayed occurrence of fatal neoplastic diseases in ames dwarf mice: correlation to extended longevity. J Gerontol A Biol Sci Med Sci, 2003. 58(4): p. 291-6.
52. Besson, A., et al., Reduced longevity in untreated patients with isolated growth hormone deficiency. J Clin Endocrinol Metab, 2003. 88(8): p. 3664-7.
53. Weiss, E.P., et al., Lower extremity muscle size and strength and aerobic capacity decrease with caloric restriction but not with exercise-induced weight loss. J Appl Physiol, 2007. 102(2): p. 634-40.
54. Dirks, A.J. and C. Leeuwenburgh, Caloric restriction in humans: potential pitfalls and health concerns. Mech Ageing Dev, 2006. 127(1): p. 1-7.
55. Soare, A., et al., Long-term calorie restriction, but not endurance exercise, lowers core body temperature in humans. Aging (Albany NY), 2011. 3(4): p. 374-9.
56. Heilbronn, L.K., et al., Effect of 6-month calorie restriction on biomarkers of longevity, metabolic adaptation, and oxidative stress in overweight individuals: a randomized controlled trial. JAMA, 2006. 295(13): p. 1539-48.
57. Cangemi, R., et al., Long-term effects of calorie restriction on serum sex-hormone concentrations in men. Aging Cell, 2010. 9(2): p. 236-42.
58. Fontana, L., et al., Effect of long-term calorie restriction with adequate protein and micronutrients on thyroid hormones. J Clin Endocrinol Metab, 2006. 91(8): p. 3232-5.
59. Clemmons, D.R. and L.E. Underwood, Nutritional regulation of IGF-I and IGF binding proteins. Annu Rev Nutr, 1991. 11: p. 393-412.
60. Ketelslegers, J.M., et al., Nutritional regulation of the growth hormone and insulin-like growth factor-binding proteins. Horm Res, 1996. 45(3-5): p. 252-7.
61. Thissen, J.P., J.M. Ketelslegers, and L.E. Underwood, Nutritional regulation of the insulin-like growth factors. Endocr Rev, 1994. 15(1): p. 80-101.
62. Fontana, L., et al., Long-term effects of calorie or protein restriction on serum IGF-1 and IGFBP-3 concentration in humans. Aging Cell, 2008. 7(5): p. 681-7.
63. Fontana, L., et al., Effects of 2-year calorie restriction on circulating levels of IGF-1, IGF-binding proteins and cortisol in nonobese men and women: a randomized clinical trial. Aging Cell, 2016. 15(1): p. 22-7.
64. Masoro, E.J. and S.N. Austad, The evolution of the antiaging action of dietary restriction: a hypothesis. J Gerontol A Biol Sci Med Sci, 1996. 51(6): p. B387-91.
65. Bathalon, G.P., et al., Metabolic, psychological, and health correlates of dietary restraint in healthy postmenopausal women. J Gerontol A Biol Sci Med Sci, 2001. 56(4): p. M206-11.
66. Goran, M.I., et al., Effects of increased energy intake and/or physical activity on energy expenditure in young healthy men. J Appl Physiol, 1994. 77(1): p. 366-72.
67. Bullough, R.C., et al., Interaction of acute changes in exercise energy expenditure and energy intake on resting metabolic rate. Am J Clin Nutr, 1995. 61(3): p. 473-81.
68. Bell, C., et al., High energy flux mediates the tonically augmented beta-adrenergic support of resting metabolic rate in habitually exercising older adults. J Clin Endocrinol Metab, 2004. 89(7): p. 3573-8.
69. Astrup, A., et al., Low resting metabolic rate in subjects predisposed to obesity: a role for thyroid status. Am J Clin Nutr, 1996. 63(6): p. 879-83.
70. Ravussin, E., et al., Reduced rate of energy expenditure as a risk factor for body-weight gain. N Engl J Med, 1988. 318(8): p. 467-72.
71. van Pelt, R.E., et al., Age-related decline in RMR in physically active men: relation to exercise volume and energy intake. Am J Physiol Endocrinol Metab, 2001. 281(3): p. E633-9.
72. Manini, T.M., et al., Daily activity energy expenditure and mortality among older adults. JAMA, 2006. 296(2): p. 171-9.
73. Paffenbarger, R.S., Jr., et al., The association of changes in physical-activity level and other lifestyle characteristics with mortality among men. N Engl J Med, 1993. 328(8): p. 538-45.
74. Lawson, E.A., et al., Appetite-regulating hormones cortisol and peptide YY are associated with disordered eating psychopathology, independent of body mass index. Eur J Endocrinol, 2011. 164(2): p. 253-61.
75. Ferrando, A.A., et al., Inactivity amplifies the catabolic response of skeletal muscle to cortisol. J Clin Endocrinol Metab, 1999. 84(10): p. 3515-21.
76. Degens, H., The role of systemic inflammation in age-related muscle weakness and wasting. Scand J Med Sci Sports, 2010. 20(1): p. 28-38.
77. Evans, W.J. and W.W. Campbell, Sarcopenia and age-related changes in body composition and functional capacity. J Nutr, 1993. 123(2 Suppl): p. 465-8.
78. Hawkins, S.A., R.A. Wiswell, and T.J. Marcell, Exercise and the master athlete--a model of successful aging? J Gerontol A Biol Sci Med Sci, 2003. 58(11): p. 1009-11.
79. Marcell, T.J., Sarcopenia: causes, consequences, and preventions. J Gerontol A Biol Sci Med Sci, 2003. 58(10): p. M911-6.
80. Reed, R.L., et al., The relationship between muscle mass and muscle strength in the elderly. J Am Geriatr Soc, 1991. 39(6): p. 555-61.
81. Roubenoff, R., Sarcopenia and its implications for the elderly. Eur J Clin Nutr, 2000. 54 Suppl 3: p. S40-7.
82. Smith, D.L., Jr., T.R. Nagy, and D.B. Allison, Calorie restriction: what recent results suggest for the future of ageing research. Eur J Clin Invest, 2010. 40(5): p. 440-50.
83. Heilbronn, L.K. and E. Ravussin, Calorie restriction and aging: review of the literature and implications for studies in humans. Am J Clin Nutr, 2003. 78(3): p. 361-9.
84. Kagawa, Y., Impact of Westernization on the nutrition of Japanese: changes in physique, cancer, longevity and centenarians. Prev Med, 1978. 7(2): p. 205-17.
85. Okabe, T., et al., Antiatherosclerotic function of Kokuto, Okinawan noncentrifugal cane sugar. J Agric Food Chem, 2009. 57(1): p. 69-75.
86. Sho, H., History and characteristics of Okinawan longevity food. Asia Pac J Clin Nutr, 2001. 10(2): p. 159-64.
87. Willcox, D.C., et al., The Okinawan diet: health implications of a low-calorie, nutrient-dense, antioxidant-rich dietary pattern low in glycemic load. J Am Coll Nutr, 2009. 28 Suppl: p. 500S-516S.
88. Salen, P. and M. de Lorgeril, The Okinawan diet: a modern view of an ancestral healthy lifestyle. World Rev Nutr Diet, 2011. 102: p. 114-23.
89. Rubner, M.,Das problem der lebensdauer. 1908, Munich: Oldenburg.
90. Ramsey, J.J., M.E. Harper, and R. Weindruch, Restriction of energy intake, energy expenditure, and aging. Free Radic Biol Med, 2000. 29(10): p. 946-68.
91. Austad, S.N. and K.E. Fischer, Mammalian aging, metabolism, and ecology: evidence from the bats and marsupials. J Gerontol, 1991. 46(2): p. B47-53.
92. Holloszy, J.O. and E.K. Smith, Longevity of cold-exposed rats: a reevaluation of the "rate-of-living theory". J Appl Physiol, 1986. 61(5): p. 1656-60.
93. McCarter, R., E.J. Masoro, and B.P. Yu, Does food restriction retard aging by reducing the metabolic rate? Am J Physiol, 1985. 248(4 Pt 1): p. E488-90.
94. Koubova, J. and L. Guarente, How does calorie restriction work? Genes Dev, 2003. 17(3): p. 313-21.
95. Lints, F.A., The rate of living theory revisited. Gerontology, 1989. 35(1): p. 36-57.
96. Manini, T.M., et al., Activity energy expenditure and mobility limitation in older adults: differential associations by sex. Am J Epidemiol, 2009. 169(12): p. 1507-16.
97. Byberg, L., et al., Total mortality after changes in leisure time physical activity in 50 year old men: 35 year follow-up of population based cohort. BMJ, 2009. 338: p. b688.
98. Castillo-Garzon, M.J., et al., Anti-aging therapy through fitness enhancement. Clin Interv Aging, 2006. 1(3): p. 213-20.
99. Lanza, I.R., et al., Endurance exercise as a countermeasure for aging. Diabetes, 2008. 57(11): p. 2933-42.
100. Huffman, D.M. and N. Barzilai, Role of visceral adipose tissue in aging. Biochim Biophys Acta, 2009. 1790(10): p. 1117-23.
101. Gabriely, I., et al., Removal of visceral fat prevents insulin resistance and glucose intolerance of aging: an adipokine-mediated process? Diabetes, 2002. 51(10): p. 2951-8.
102. Ohkawara, K., et al., A dose-response relation between aerobic exercise and visceral fat reduction: systematic review of clinical trials. Int J Obes (Lond), 2007. 31(12): p. 1786-97.
103. Fischer, K., et al., Association of Habitual Patterns and Types of Physical Activity and Inactivity with MRI-Determined Total Volumes of Visceral and Subcutaneous Abdominal Adipose Tissue in a General White Population. PLoS One, 2015. 10(11): p. e0143925.
104. Redman, L.M., et al., Effect of calorie restriction with or without exercise on body composition and fat distribution. J Clin Endocrinol Metab, 2007. 92(3): p. 865-72.
105. Ingram, D.K. and G.S. Roth, Calorie restriction mimetics: can you have your cake and eat it, too? Ageing Res Rev, 2015. 20: p. 46-62.
106. Gillespie, Z.E., J. Pickering, and C.H. Eskiw, Better Living through Chemistry: Caloric Restriction (CR) and CR Mimetics Alter Genome Function to Promote Increased Health and Lifespan. Front Genet, 2016. 7: p. 142.
107. Chen, D. and L. Guarente, SIR2: a potential target for calorie restriction mimetics. Trends Mol Med, 2007. 13(2): p. 64-71.
108. Ingram, D.K., et al., Development of calorie restriction mimetics as a prolongevity strategy. Ann N Y Acad Sci, 2004. 1019: p. 412-23.
109. Ingram, D.K. and G.S. Roth, Glycolytic inhibition as a strategy for developing calorie restriction mimetics. Exp Gerontol, 2011. 46(2-3): p. 148-54.
110. Ingram, D.K., et al., Calorie restriction mimetics: an emerging research field. Aging Cell, 2006. 5(2): p. 97-108.
111. Roth, G.S., M.A. Lane, and D.K. Ingram, Caloric restriction mimetics: the next phase. Ann N Y Acad Sci, 2005. 1057: p. 365-71.
112. Handschin, C., Caloric restriction and exercise "mimetics'': Ready for prime time? Pharmacol Res, 2016. 103: p. 158-66.
113. Barzilai, N. and A. Bartke, Biological approaches to mechanistically understand the healthy life span extension achieved by calorie restriction and modulation of hormones. J Gerontol A Biol Sci Med Sci, 2009. 64(2): p. 187-91.
114. Fraser, G.E. and D.J. Shavlik, Ten years of life: Is it a matter of choice? Arch Intern Med, 2001. 161(13): p. 1645-52.
115. Booth, F.W., C.K. Roberts, and M.J. Laye, Lack of exercise is a major cause of chronic diseases. Compr Physiol, 2012. 2(2): p. 1143-211.
116. Booth, F.W., M.J. Laye, and M.D. Roberts, Lifetime sedentary living accelerates some aspects of secondary aging. J Appl Physiol (1985), 2011. 111(5): p. 1497-504.
117. Fries, J.F., B. Bruce, and E. Chakravarty, Compression of morbidity 1980-2011: a focused review of paradigms and progress. J Aging Res, 2011. 2011: p. 261702.
118. Lee, D., et al., Effects of nutritional components on aging. Aging Cell, 2015. 14(1): p. 8-16.
119. Kassi, E. and A.G. Papavassiliou, Could glucose be a proaging factor? J Cell Mol Med, 2008. 12(4): p. 1194-8.
120. Rosedale, R., E.C. Westman, and J.P. Konhilas, Clinical Experience of a Diet Designed to Reduce Aging. J Appl Res, 2009. 9(4): p. 159-165.
121. Shanley, D.P. and T.B. Kirkwood, Caloric restriction does not enhance longevity in all species and is unlikely to do so in humans. Biogerontology, 2006. 7(3): p. 165-8.
122. Hopkins, M. and J.E. Blundell, Energy balance, body composition, sedentariness and appetite regulation: pathways to obesity. Clin Sci (Lond), 2016. 130(18): p. 1615-28.
123. Cameron, J.D., et al., Energy depletion by diet or aerobic exercise alone: impact of energy deficit modality on appetite parameters. Am J Clin Nutr, 2016. 103(4): p. 1008-16.
124. Blundell, J.E., et al., Appetite control and energy balance: impact of exercise. Obes Rev, 2015. 16 Suppl 1: p. 67-76.
125. Shook, R.P., et al., Low levels of physical activity are associated with dysregulation of energy intake and fat mass gain over 1 year. Am J Clin Nutr, 2015. 102(6): p. 1332-8.